Temperature Effects on Growth Rates and Predation Loss of Bacterial Groups in Marine Ecosystems
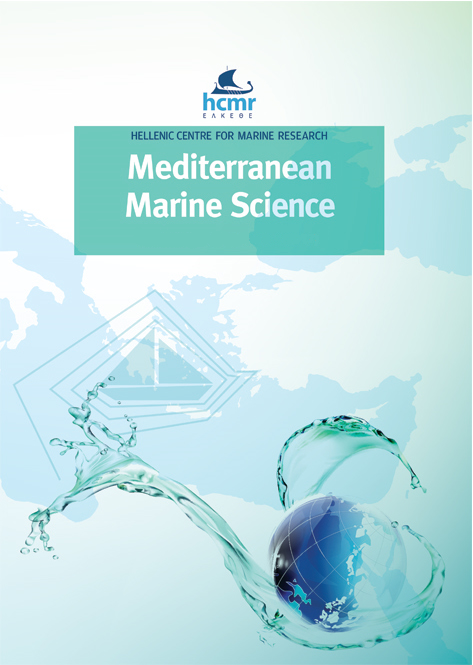
Abstract
This study investigated the effects of experimental warming on the major bacterial phylogenetic groups in the marine environment, focusing on their growth and grazing rates, phylogenetic community composition and grazing preferences. A series of growth and grazing experiments were performed in microcosms at a temperature increase of 3°C. The growth rates of all bacterial groups were enhanced by temperature increase. SAR11 and Roseobacter were particularly sensitive to temperature changes, while Gammaproteobacteria were the least affected. Protozoan grazing mainly affected fast-growing groups such as Roseobacter and Gammaproteobacteria, while slower-growing groups such as SAR11 were less affected. In most cases, the growth rates of the bacteria increased more with temperature than the grazing rates of predators. This indicates that temperature has a stronger influence on bacterial growth than on predator activity. At higher temperatures the grazing pressure of nanoflagellates (NF) on Roseobacter and Bacteroidetes increased while Gammaproteobacteria were almost exclusively grazed by ciliates (CIL). As a result, the dominant predator of certain groups and the composition of prey changed with temperature increase. The changes in bacterial community composition at higher temperatures were caused both by the direct effect of temperature on bacterial growth and by shifts in predator grazing preferences. Furthermore, our results suggest that bacterial growth and grazing behaviour of bacterial predators do not necessarily occur simultaneously at higher temperatures and highlight the importance of considering bacterial phylogenetic composition when predicting bacterial response to warming.
Article Details
- How to Cite
-
ŠESTANOVIĆ, S., VRDOLJAK TOMAŠ, A., ŠOLIĆ, M., ŠANTIĆ, D., & BOJANIĆ, N. (2025). Temperature Effects on Growth Rates and Predation Loss of Bacterial Groups in Marine Ecosystems. Mediterranean Marine Science, 26(1), 131–148. https://doi.org/10.12681/mms.38158
- Section
- Research Article
Authors who publish with this journal agree to the following terms:
- Authors retain copyright and grant the journal right of first publication with the work simultaneously licensed under a Creative Commons Attribution Non-Commercial License that allows others to share the work with an acknowledgement of the work's authorship and initial publication in this journal.
- Authors are able to enter into separate, additional contractual arrangements for the non-exclusive distribution of the journal's published version of the work (e.g. post it to an institutional repository or publish it in a book), with an acknowledgement of its initial publication in this journal.
- Authors are permitted and encouraged to post their work online (preferably in institutional repositories or on their website) prior to and during the submission process, as it can lead to productive exchanges, as well as earlier and greater citation of published work (See The Effect of Open Access).